-by David Németh.
Gene Drives
Gene drives increase the inheritance of alleles, even if they do not help the organism against natural selection or in reproduction. Gene drives can be used in populations that reproduce by sexual reproduction The spread of Gene Drives is limited by generation time. It is important to know the ecological, ethical and social risks of gene drives.
Ecological problems: gene drives can alter or suppress the genome of a wild population, which can lead to changes in natural, native populations. Ecological problems can change depending on the gene drive used and the target organism, e.g., by spreading the gene drive indefinitely or disrupting the balance of food chains or food webs by reducing the number of primary and/or secondary consumers or by altering the genome of native producers.
Ethical risks include using gene drives to suppress and eradicate certain populations or using gene drives to alter entire populations for our own benefit. Social risks can occur after an accident or failure of a gene driver, which can lead to lack of foundation or loss of trust. In more serious cases, gene drivers may be banned (von Gleich, 2020; ,Deplazes et al., 2020; Noble et al., 2019; Roberts et al., 2022).
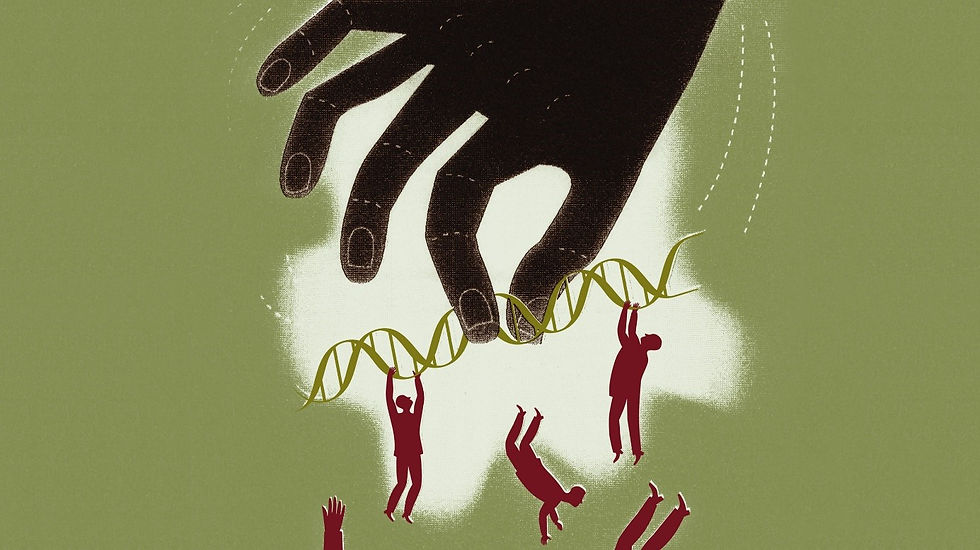
Source: QuantaMagazine
Uses of specific gene dives and the difference between them
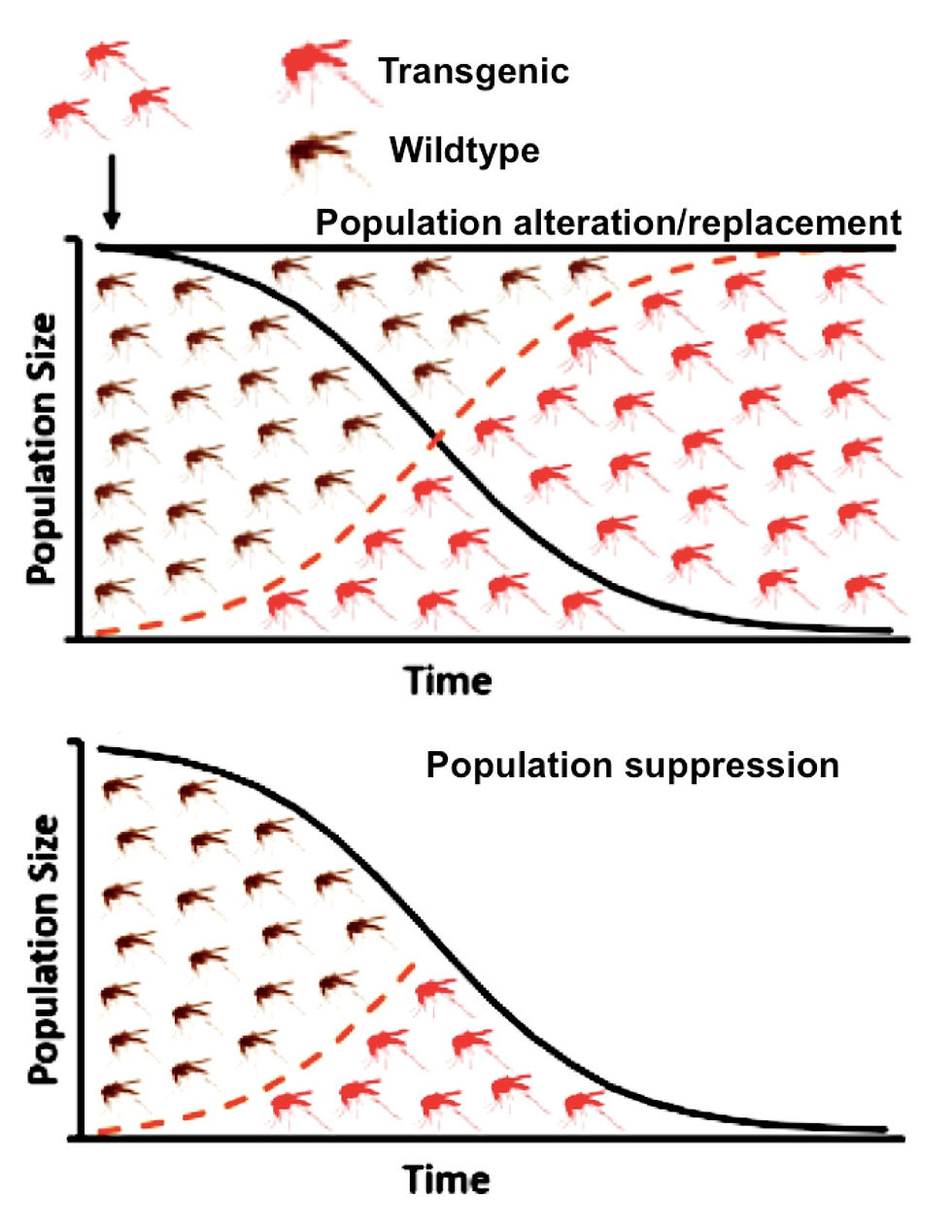
Population alteration gene drives allow us to delete specific genes from the genome of wild populations (Esvelt et al., 2014; Stephanie et al. 2018). This method is reliable because the target gene is either replaced or deleted completely from the genome.
Population alteration gene drives can also be used to add or edit certain genes, but this method is not as reliable because mutations can occur in the transferred gene, so this method is not evolutionarily stable (Champer et al., 2021). On the other hand, we can suppress wild populations with suppression gene drives (Curtis, 1968), which can be achieved with various strategies, like female fertility homing drive or Both-sex fertility homing drive.
Source: Haylab
Local gene drives
Local gene drives use chromosomal translocations to produce two transposed wild type chromosomes by breaking of one arm of each chromosome and swapping them the result is an organism that has two copies of all genes, but after reproducing with a wild organism, there are four potential chromosome arrangements, one of which contains two wild- type chromosomes, the other two translocated chromosomes, and the other two chromosome arrangements contain one wild chromosome and one translocated chromosome - these hybrids lack important genetic information, so in most cases they are lethal.
Local gene drive are very weak genetic engineering tools, which means that the spread of these drives is very limited.
To use them effectively, one must release more engineered organisms than the living wild population (DiCarlo et al., 2015).
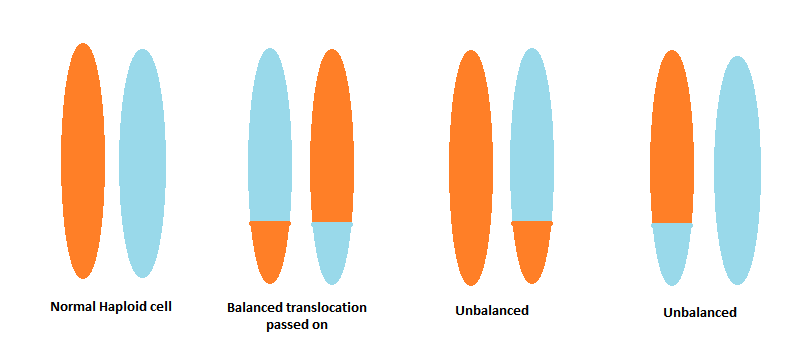
Source: www.almostadoctor.com
Standard gene drives
Standard gene drives, also called self-propagating drives ( Okamoto et al., 2014; Noble et al., 2018) , work by increasing the probability of allele inheritance from 50% to 100% by using CRISPR (Deplazes et al., 2020) to create a double-strand break at the target allele and force the organism to fix the cut either by homologous recombination or by non-homologous end joining. In homologous recombination, our drive is cloned into the allele, increasing inheritance to 100%.
In non-homologous end joining, the allele is deleted. In this way, inheritance of the drive remains the same, but there is a missing allele in the other 50%. To avoid non-homologous end joining, most standard gene drives are located near important genes, so the pressure of natural selection favors organisms with intact drives.
With standard gene drives, it is difficult to control the spread of the gene drive. Mutations can lead to changes in the genes of our drive. Gene drives can spread to other closely related species by hybridization and in this way affect other native species.
Reproductive hybrids in animals are very rare, but plants can often produce reproductive hybrid organisms. Resistant alleles can occur in the wild population, which means that CRISPR cannot generate the double strand break (Champer et al., 2017).
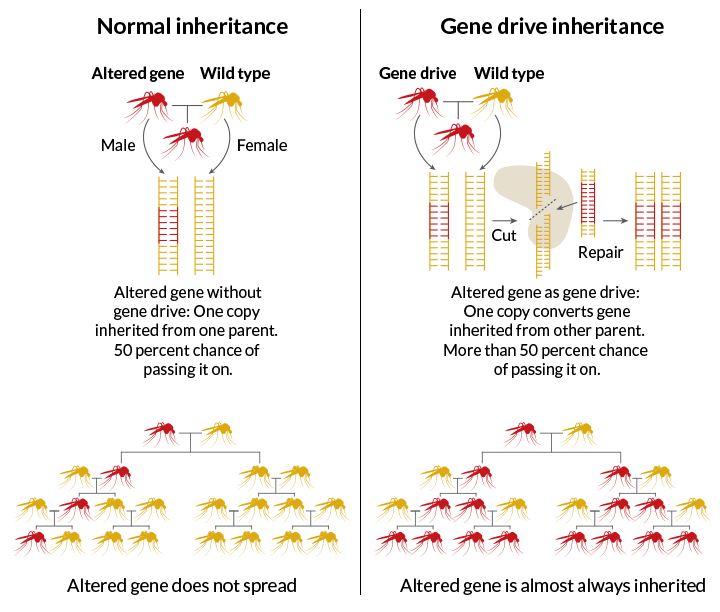
Source: Science news
Daisy-chain gene drives
Daisy chains work on the principle of standard gene drives. Daisy chains are a much safer alternative to a standard gene drive. The daisy chain drive achieves this by different daisy elements are distributed on different chromosomes: Element C intersects the allele next to element B, so element B can be cloned there, and element B intersects the allele next to element A, so element A can be cloned there, but C cannot be cloned, so only 50% of the offspring inherit element C of the daisy chain.
This way, controlled spread can be achieved. The spread of the drive can be controlled by the number of drives released into the wild population. One can increase the spread of the daisy drive by increasing the number of elements, which results in the drive being passed on to more generations (DiCarlo et al., 2015; Noble et al., 2019).
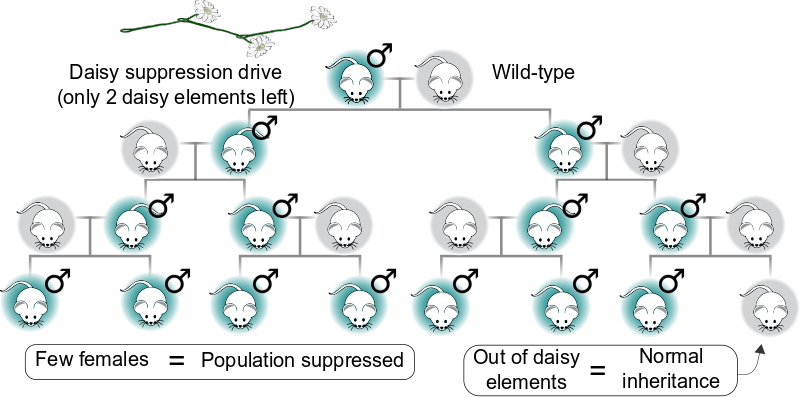
Source: News MIT
References
1. Curtis C. (1968). Possible Use of Translocations to fix Desirable Genes in Insect Pest Populations. Nature 218, 368–369 (1968). https://doi.org/10.1038/218368a0
2. von Gleich, Arnim. (2020) "Steps Towards a Precautionary Risk Governance of SPAGE Technologies Including Gene-Drives." Gene Drives at Tipping Points.
3. Charleston Noble, Ben Adlam, George M Church, Kevin M Esvelt, Martin A Nowak (2018) Current CRISPR gene drive systems are likely to be highly invasive in wild populations. https://doi.org/10.7554/eLife.33423
4. Champer, J., Kim, I.K., Champer, S.E., Clark, A.G. and Messer, P.W. (2021), Suppression gene drive in continuous space can result in unstable persistence of both drive and wild-type alleles. Mol Ecol, 30: 1086-1101. https://doi.org/10.1111/mec.15788
5. Deplazes Zemp, Anna & Lefort, François & Müller, Pie & Romeis, Jörg & Rüegsegger, Adrian & Schoenenberger, Nicola & Spehn, Eva. (2020). Gene drives: benefits, risks, and possible applications. 15. 1-7. 10.5281/zenodo.3742771.
6. Charleston Noble, John Min, Jason Olejarz, Joanna Buchthal, Alejandro Chavez, Andrea L. Smidler, Erika A. DeBenedictis, George M. Church, Martin A. Nowak, Kevin M. Esvelt (2019),Daisy-chain gene drives for the alteration of local populations bioRxiv 057307; doi: https://doi.org/10.1101/057307
7. DiCarlo, J., Chavez, A., Dietz, S. et al. Safeguarding CRISPR-Cas9 gene drives in yeast. Nat Biotechnol 33, 1250–1255 (2015). https://doi.org/10.1038/nbt.3412
8. Champer J, Reeves R, Oh SY, Liu C, Liu J, et al. (2017) Novel CRISPR/Cas9 gene drive constructs reveal insights into mechanisms of resistance allele formation and drive efficiency in genetically diverse populations. PLOS Genetics 13(7): e1006796. https://doi.org/10.1371/journal.pgen.1006796
9. Noble, C., Min, J., Olejarz, J., Buchthal, J., Chavez, A., Smidler, A. L., DeBenedictis, E. A., Church, G. M., Nowak, M. A., & Esvelt, K. M. (2019). Daisy-chain gene drives for the alteration of local populations. Proceedings of the National Academy of Sciences, 116(17), 8275–8282. https://doi.org/10.1073/pnas.1716358116
10. Okamoto, Kenichi W., et al. "Feasible introgression of an anti-pathogen transgene into an urban mosquito population without using gene-drive." PLoS neglected tropical diseases 8.7 (2014): e2827.
11. James, Stephanie et al. “Pathway to Deployment of Gene Drive Mosquitoes as a Potential Biocontrol Tool for Elimination of Malaria in Sub-Saharan Africa: Recommendations of a Scientific Working Group†.” The American journal of tropical medicine and hygiene vol. 98,6_Suppl (2018): 1-49. doi:10.4269/ajtmh.18-0083
12. Roberts, A.J., Thizy, D. Articulating ethical principles guiding Target Malaria's engagement strategy. Malar J 21, 35 (2022). https://doi.org/10.1186/s12936-022-04062-4
Комментарии